We highly recommend the top ten techniques for atmospheric condition analysis using advanced technologies and data-driven methods. Satellite imagery offers high-resolution data for monitoring weather patterns. Doppler radar measures wind speeds and detects storm formations. Weather balloons collect real-time upper-atmospheric data. Remote sensing provides global atmospheric monitoring. LIDAR technology maps cloud structures, particulate concentrations, and wind patterns. Atmospheric sounding profiles temperature and humidity at various altitudes. Computer models predict weather phenomena. Wind profilers measure wind speed and direction. Lightning detection systems track and analyze storm intensity. Together, these tools offer thorough insights for robust weather forecasting. Discover these methodologies' intricate details for optimized atmospheric analysis.
Key Points
- Satellite imagery offers high-resolution data for monitoring weather patterns and tracking storm systems globally.
- Doppler radar measures wind speed and direction, enhancing weather forecasting accuracy and tornado detection.
- Weather balloons collect real-time upper-atmosphere data on temperature, humidity, pressure, and wind speeds.
- Remote sensing provides global coverage for tracking environmental variables and phenomena like hurricanes and droughts.
Satellite Imagery
Satellite imagery provides us with a powerful tool for analyzing atmospheric conditions by delivering high-resolution data from space-based sensors. By leveraging satellite imagery interpretation, we can monitor weather patterns, track storm systems, and observe cloud formations with exceptional detail. This capability allows us to perform accurate weather monitoring, which is pivotal for predicting severe weather events and making informed decisions that protect lives and property.
When we engage in atmospheric data analysis, we uncover valuable insights into climate trends. Satellite data helps us to measure variables such as temperature, humidity, and atmospheric pressure over large areas and extended periods. This extensive view enables us to detect shifts in climate patterns, identify long-term trends, and understand the underlying causes of climate change.
Moreover, the integration of satellite imagery with advanced algorithms enhances our ability to process and interpret vast amounts of data efficiently. By doing so, we gain a clearer picture of the Earth's atmospheric dynamics, empowering us to develop more precise models and forecasts.
In essence, satellite imagery is essential for atmospheric condition analysis. It provides us with the data-driven precision necessary to monitor weather and analyze climate trends, enabling us to make informed, autonomous decisions in a rapidly changing world.
Doppler Radar
Let's explore how Doppler Radar operates, focusing on the principles that enable it to measure velocity and precipitation intensity.
We'll discuss data interpretation methods that transform raw radar signals into actionable meteorological insights.
Principles of Doppler Radar
Doppler radar, a pivotal tool in meteorology, measures the velocity of particles in the atmosphere by analyzing the frequency shift of returned radar signals. This capability allows us to detect wind speed and direction, which is essential for weather forecasting and severe storm tracking.
Among the myriad Doppler radar applications, the system excels in identifying rotational patterns within storms, critical for tornado warnings, and in detecting precipitation intensity, aiding in flood forecasting.
However, the technology isn't without limitations. Doppler radar can struggle with range folding, where distant signals overlap with closer ones, and it's less effective in mountainous terrain due to signal obstruction. Additionally, it sometimes has difficulty differentiating between precipitation types, such as rain versus hail.
On the bright side, Doppler radar technology advancements continue to enhance our atmospheric analysis capabilities. Dual-polarization radars, for example, transmit and receive both horizontal and vertical pulses, providing more detailed data on precipitation shape and size. This advancement improves accuracy in distinguishing between different types of precipitation and even non-meteorological objects, like birds or debris.
Data Interpretation Methods
To effectively interpret Doppler radar data, we must utilize advanced algorithms that process velocity and reflectivity information to generate accurate meteorological insights. By employing statistical analysis, we can discern patterns and anomalies in atmospheric conditions, which are critical for understanding weather phenomena. These algorithms help us convert raw data into meaningful visual representations, enabling us to grasp complex atmospheric dynamics quickly.
Real-time monitoring is indispensable for immediate weather assessments. Through continuous data collection and processing, we get an up-to-the-minute view of current conditions, allowing us to respond swiftly to emerging weather events. Additionally, trend analysis derived from Doppler radar data helps us identify long-term patterns and shifts in atmospheric behavior, providing a thorough understanding of weather trends over time.
Our approach leverages high-resolution visual representation tools, which facilitate the clear depiction of velocity fields and precipitation intensity. These visual tools not only enhance our immediate comprehension but also empower us to communicate findings effectively to a broader audience.
Enhancing Forecast Accuracy
By leveraging Doppler radar technology, we greatly enhance forecast accuracy through precise measurement of wind velocity and precipitation patterns. This advanced radar system allows us to detect minute changes in weather conditions, providing data that's critical for accurate predictions.
The integration of machine learning applications further refines our forecasting models by analyzing vast datasets and detecting complex patterns that might be missed through traditional methods.
We use sophisticated visualization techniques to present Doppler radar data in a way that's both intuitive and informative. These techniques transform raw data into comprehensible formats, enabling us to make quick, informed decisions. For instance, color-coded velocity maps and three-dimensional precipitation models allow us to pinpoint areas of severe weather with high precision.
Machine learning algorithms continuously improve as more data becomes available, enhancing their predictive capabilities over time. This iterative learning process allows for real-time adjustments, making our forecasts increasingly reliable.
The combination of Doppler radar technology and machine learning applications empowers us to deliver timely and accurate weather forecasts, giving individuals and communities the freedom to plan and respond effectively to atmospheric conditions. By implementing these advanced technologies, we set a new standard in meteorological forecasting.
Weather Balloons
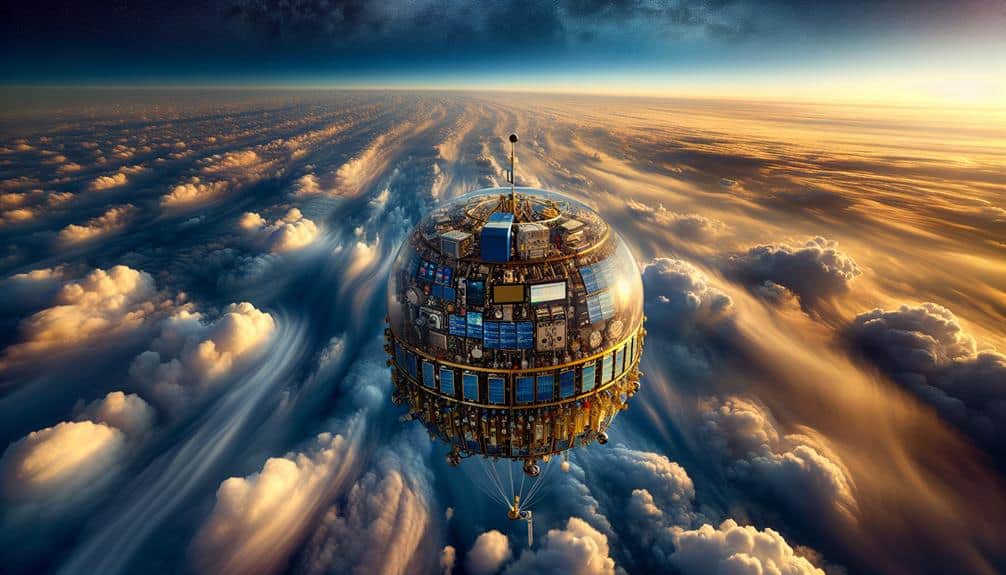
Weather balloons, also known as sounding balloons, play an important role in collecting upper-atmosphere data necessary for precise weather forecasting. By ascending through the atmosphere, they gather real-time data on temperature, humidity, pressure, and wind speeds at various altitudes. This information is essential for constructing accurate weather models that enhance our ability to predict meteorological events.
Let's explore balloon trajectory analysis. Understanding a balloon's path is essential for interpreting the data it collects. By analyzing trajectory, we can consider factors such as wind patterns and atmospheric pressure changes that might influence the balloon's movement. This guarantees the data's reliability, providing meteorologists with the necessary insights to improve their forecasts.
Weather balloon technology advancements have greatly improved data collection capabilities. Modern balloons are equipped with advanced sensors and GPS systems, allowing for more precise tracking and higher-resolution data. These advancements enable us to gather detailed atmospheric profiles, which are necessary for understanding complex weather phenomena and enhancing predictive accuracy.
Remote Sensing
Remote sensing utilizes satellite and airborne instruments to collect extensive atmospheric data, which is vital for analyzing weather patterns and climate dynamics. By leveraging these advanced technologies, we can monitor a wide array of environmental variables, including temperature, humidity, and wind speed, over vast geographical areas. This capability is instrumental in both short-term weather forecasting and long-term climate studies.
Among the myriad remote sensing applications, satellite-based sensors provide continuous, global coverage, allowing us to track and predict phenomena like hurricanes, droughts, and heatwaves with high precision. Airborne sensors, on the other hand, offer high-resolution data over smaller, targeted regions, making them ideal for localized studies and emergency response scenarios.
Data interpretation techniques play a pivotal role in translating raw sensor data into actionable insights. Methods such as spectral analysis, radiometric calibration, and geospatial mapping enable us to derive meaningful patterns and trends from the collected information. For instance, spectral analysis helps in identifying specific atmospheric components by examining their unique spectral signatures.
LIDAR Technology
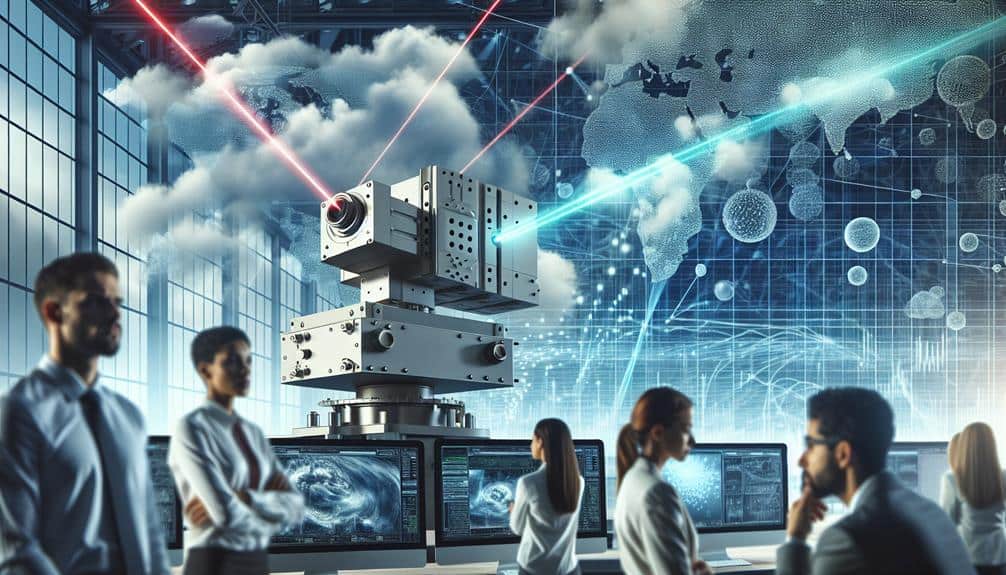
Let's explore how LIDAR technology enables us to measure atmospheric particulates with high precision, analyze wind patterns for meteorological studies, and map cloud structures in three dimensions.
By emitting laser pulses and measuring their return time, we gather detailed data on particulate concentration, airflow dynamics, and cloud formation.
This data-driven approach enhances our ability to predict weather patterns and understand atmospheric processes.
Measuring Atmospheric Particulates
Utilizing Light Detection and Ranging (LIDAR) technology, we can accurately measure atmospheric particulates by emitting laser pulses and analyzing the scattered light. This allows us to obtain high-resolution data on particulate matter distribution, which is vital for air quality assessment and pollution monitoring. LIDAR's ability to penetrate various atmospheric layers provides a detailed vertical profile of particulate concentration, enabling us to identify pollution sources and track their dispersion.
Using LIDAR, we can quantify particulate matter with remarkable precision, detecting even slight changes in atmospheric composition. This technology's capacity to generate real-time data makes it invaluable for immediate air quality assessments and long-term environmental studies. By integrating LIDAR measurements with ground-based sensors, we can enhance the accuracy of our pollution monitoring systems, ensuring that we capture a holistic view of airborne particulates.
Furthermore, LIDAR's adaptability to different wavelengths enables us to differentiate between various types of particulates, such as dust, smoke, and industrial emissions. This specificity helps us develop targeted strategies for mitigating pollution and improving public health.
Analyzing Wind Patterns
By utilizing LIDAR technology, we can precisely analyze wind patterns by emitting laser pulses and measuring the Doppler shift in the returned signal. This method allows us to accurately capture data vital for wind speed analysis and turbulence detection. LIDAR's high-resolution capabilities enable us to dissect both seasonal wind patterns and local wind effects, offering a thorough understanding of atmospheric dynamics.
Here's how LIDAR technology helps us analyze wind patterns:
- Wind Speed Analysis: By evaluating the Doppler shift of the reflected laser signals, we can measure the speed and direction of wind with high accuracy. This data is essential for understanding wind behavior at various altitudes.
- Turbulence Detection: LIDAR enables us to detect small-scale turbulence, providing insights into atmospheric stability and potential impacts on aviation and weather forecasting.
- Seasonal Wind Patterns: Our ability to monitor how wind patterns change with seasons helps us predict long-term weather trends and assess climate models more accurately.
- Local Wind Effects: By focusing on specific geographic areas, we can analyze how local topography and urban structures influence wind behavior, aiding in urban planning and environmental assessments.
Harnessing LIDAR technology empowers us to gain precise, data-driven insights into the ever-changing wind patterns that shape our environment.
Cloud Structure Mapping
LIDAR technology allows us to map cloud structures with exceptional precision, capturing detailed data on cloud density, altitude, and layering. By emitting laser pulses and measuring their return times, LIDAR provides high-resolution vertical profiles of cloud height. This technology excels in identifying cloud base and top altitudes, essential for accurate precipitation forecasting.
When we deploy LIDAR systems, we can penetrate multiple cloud layers, distinguishing between stratiform and cumuliform structures with ease. The data collected informs us about the microphysical properties of clouds, such as droplet size distribution and liquid water content. These parameters are crucial for understanding and predicting precipitation patterns.
Moreover, integrating LIDAR data with satellite observations and ground-based radar enhances our analytical capabilities. We obtain a thorough 3D view of cloud dynamics, enabling us to assess cloud evolution over time. This multi-faceted approach improves our ability to predict severe weather events and optimize flight paths for aviation safety.
Surface Observations
Surface observations form the backbone of atmospheric condition analysis, providing necessary real-time data on temperature, humidity, wind speed, and atmospheric pressure. By examining temperature trends and humidity levels, we can gain a thorough understanding of the local climate and predict weather patterns with greater accuracy.
Tracking these parameters helps us identify anomalies and shifts that might indicate larger atmospheric changes.
Monitoring air pressure variations is vital for forecasting weather events like storms or calm periods. Changes in atmospheric pressure often precede significant weather changes, giving us valuable lead time to prepare.
Precipitation rates, another essential metric, inform us about the likelihood and intensity of rainfall, snow, or other forms of precipitation, which is crucial for agriculture, water resource management, and disaster preparedness.
To effectively utilize surface observations, we should focus on:
- Establishing a network of reliable weather stations: Ensuring data consistency and coverage.
- Utilizing high-precision instruments: For accurate readings of temperature, humidity, air pressure, and precipitation.
- Implementing automated data collection: For real-time analysis and faster decision-making.
- Integrating data with advanced modeling software: To predict future atmospheric conditions and provide actionable insights.
Atmospheric Sounding
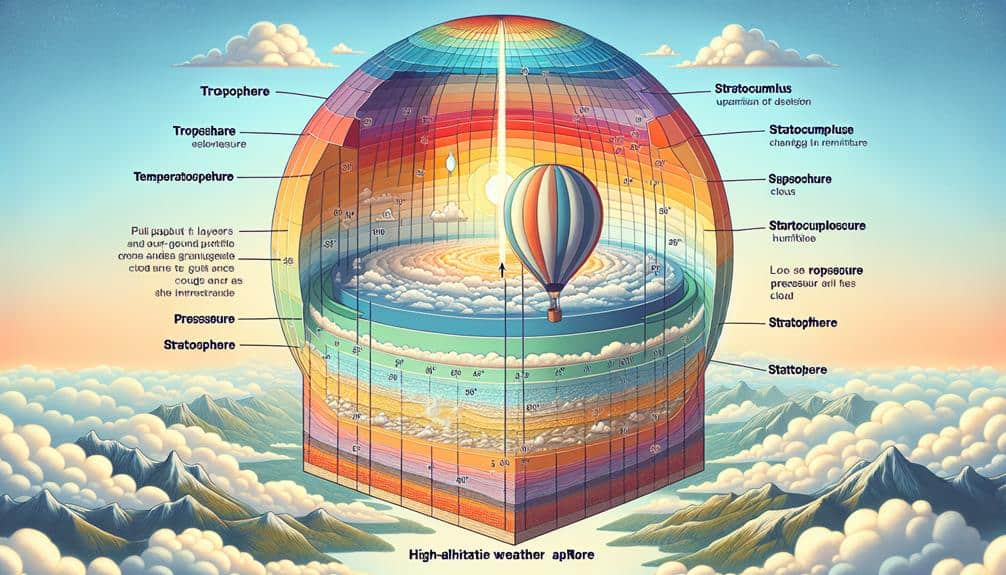
Let's explore atmospheric sounding by examining radiosonde data collection, satellite remote sensing, and weather balloon deployment.
We obtain vertical profiles of temperature, humidity, and wind through radiosondes, which provide high-resolution data.
Satellite remote sensing complements these measurements by offering expansive spatial coverage, while weather balloons enable us to gather real-time atmospheric information.
Radiosonde Data Collection
Radiosonde data collection, a vital method for atmospheric sounding, provides us with high-resolution vertical profiles of temperature, humidity, and pressure. By launching weather balloons equipped with radiosondes, we can perform balloon trajectory analysis and sonde data comparison, making sure we capture accurate upper air observations. These observations are essential for atmospheric profile generation, enhancing our comprehension of the vertical structure of the atmosphere.
To effectively utilize radiosonde data collection, we should focus on:
- Balloon Trajectory Analysis: Monitoring the path of the radiosonde allows us to correct for drift and ensure precise data capture.
- Sonde Data Comparison: Comparing data from multiple sondes launched at different times or locations offers insights into atmospheric variations and consistency.
- Upper Air Observations: Gathering data from higher altitudes helps us analyze the atmosphere's vertical structure, important for weather prediction and climate studies.
- Atmospheric Profile Generation: Creating detailed profiles of the atmosphere aids in understanding temperature gradients, humidity levels, and pressure changes over altitude.
Satellite Remote Sensing
How can we leverage satellite remote sensing to obtain extensive atmospheric soundings that complement ground-based techniques?
By utilizing satellite remote sensing, we can achieve a detailed analysis of atmospheric conditions on a global scale. First, we ensure precise satellite calibration, which is essential for accurate measurements. Well-calibrated satellites provide reliable data critical for atmospheric modeling, enhancing our understanding of weather patterns and climate dynamics.
High sensor resolution is another key factor. With advanced sensors, we capture detailed vertical profiles of temperature, humidity, and other atmospheric parameters. This high-resolution data allows us to identify and analyze fine-scale atmospheric features that ground-based methods might miss.
Efficient data processing techniques are essential for handling the vast amounts of information collected. Advanced algorithms process and integrate satellite data, enabling us to generate accurate and timely atmospheric soundings.
Weather Balloon Deployment
Deploying weather balloons equipped with sophisticated instruments allows us to obtain vertical profiles of atmospheric parameters essential for precise weather forecasting and climate research. These balloons, known as radiosondes, ascend through the atmosphere, capturing data on temperature, humidity, pressure, and wind speed.
Here's how we optimize weather balloon deployment for accurate atmospheric sounding:
- Balloon Tracking: We employ GPS technology for precise balloon tracking. This enables us to monitor the balloon's trajectory and gather data on wind direction and speed across various altitudes.
- Data Transmission: Real-time data transmission is pivotal. Radiosondes relay information to ground stations instantaneously, allowing meteorologists to analyze evolving weather patterns without delay.
- Altitude Adjustments: By adjusting the balloon's ascent rate, we can focus on specific atmospheric layers. This flexibility is essential for studying phenomena such as temperature inversions and jet streams.
- Comprehensive Weather Analysis: Combining data from multiple balloons enhances our understanding of regional weather patterns. By launching balloons from different locations simultaneously, we build a detailed three-dimensional picture of the atmosphere.
Using these techniques, we gain a deeper insight into meteorological processes, empowering us to make informed decisions and predictions.
Weather balloon deployment is a cornerstone of atmospheric science, offering unparalleled data precision and enhancing our freedom to explore and understand our environment.
Computer Models
Computer models play an important role in simulating atmospheric conditions by integrating vast datasets and sophisticated algorithms to predict weather patterns accurately. Leveraging machine learning and advanced algorithm development, we can enhance the precision and reliability of these models.
Machine learning techniques enable the processing of massive amounts of data, identifying patterns and relationships that traditional methods might overlook. This allows for continuous improvement in forecast accuracy.
Data assimilation is vital in refining these models. By incorporating real-time observational data, we guarantee our models remain current and reflective of actual atmospheric conditions. This process integrates data from various sources, including satellites, radars, and weather stations, to create a thorough and cohesive representation of the atmosphere.
Model validation is another important aspect. We must rigorously compare model outputs with observed data to assess their accuracy and reliability. This iterative process helps us identify any discrepancies and refine our models accordingly. By continually validating and adjusting our models, we maintain their relevance and effectiveness in predicting future atmospheric conditions.
Wind Profilers
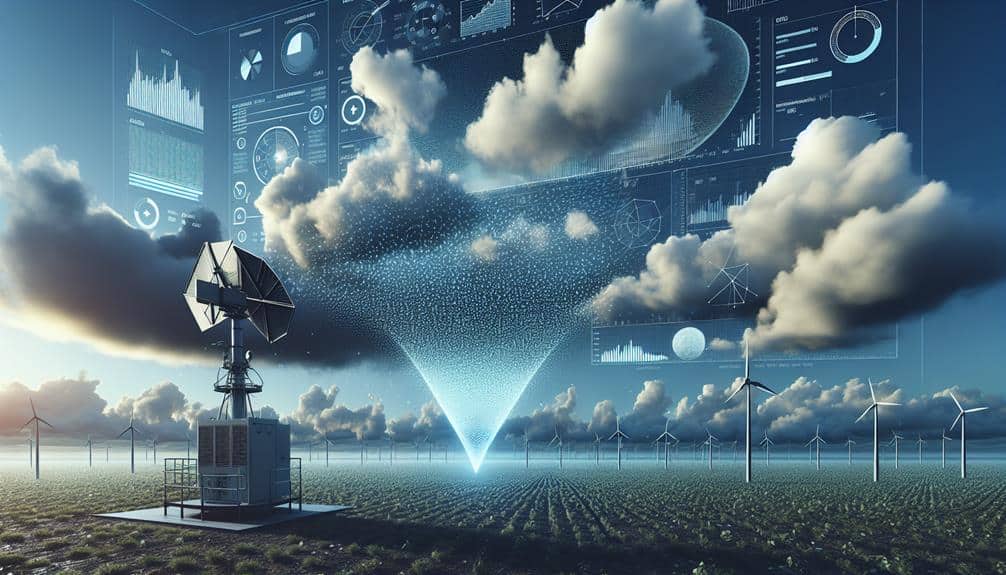
Wind profilers play a crucial role in capturing vertical profiles of wind speed and direction, providing vital observational data that complements and enhances our atmospheric computer models. By integrating data from these sophisticated instruments, we can significantly improve our forecasting accuracy, enabling us to predict weather changes with greater precision.
Recent advancements in wind profilers have revolutionized our ability to analyze atmospheric conditions. These devices use Doppler radar or acoustic sounders to measure wind components at different altitudes, offering detailed insights into wind patterns. The integration of this data into our models allows for more precise simulations of atmospheric dynamics.
The applications of wind profilers go beyond weather forecasting and are essential in various sectors, including aviation, renewable energy, and climate research.
- Aviation Safety: Enhanced wind data reduces turbulence risks and enhances flight planning.
- Renewable Energy: Accurate wind profiles assist in optimal siting and operation of wind turbines.
- Climate Studies: Long-term wind data contributes to understanding climate change patterns.
- Severe Weather Prediction: Real-time wind data aids in predicting severe weather events such as storms and hurricanes.
Lightning Detection
Lightning detection systems, leveraging advanced sensor arrays and real-time data analytics, are essential in monitoring and predicting electrical storm activity with unparalleled accuracy. These systems utilize a network of ground-based and satellite sensors to capture data on lightning strikes. By measuring the electric field, we can detect and map lightning with remarkable precision, aiding in thorough thunderstorm tracking.
Our primary tool, lightning mapping, identifies the exact locations of lightning discharges within a storm. The data collected is then processed to create a dynamic map, highlighting areas of intense electrical activity. This capability is vital for storm prediction, allowing us to forecast the development and trajectory of thunderstorms with high reliability.
Accurate storm prediction hinges on our ability to analyze the electric field variations preceding a lightning event. By scrutinizing these variations, we can anticipate lightning strikes minutes before they occur. This proactive approach not only enhances public safety but also mitigates damage to infrastructure.
Frequently Asked Questions
How Do Atmospheric Conditions Impact Air Travel Safety?
We analyze weather patterns and turbulence to guarantee air travel safety. Wind shear and visibility assessments are essential for our data-driven approach, helping us navigate atmospheric conditions and maintain freedom to travel securely and efficiently.
What Role Do Atmospheric Conditions Play in Climate Change?
We're seeing atmospheric conditions greatly influence climate change through increased greenhouse gas emissions. These emissions drive global warming trends, leading to rising temperatures, extreme weather events, and shifts in ecosystems, affecting our environment and freedom.
How Can Atmospheric Analysis Improve Agriculture?
We can enhance agriculture benefits by analyzing weather patterns. Accurate atmospheric analysis helps us predict rainfall, temperature, and extreme weather events, optimizing planting schedules and improving crop yield resilience, ultimately providing farmers greater freedom and efficiency.
What Education Is Required to Become an Atmospheric Scientist?
Did you know 60% of atmospheric scientists hold advanced degrees? We need a bachelor's in meteorology or related fields. Graduate degrees open more atmospheric research opportunities, increasing our expertise and freedom to innovate in meteorological sciences.
How Do Atmospheric Conditions Affect Human Health?
It is evident that atmospheric conditions directly impact health effects. Elevated pollution levels can worsen respiratory illnesses, while temperature fluctuations contribute to various health risks. Understanding these variables helps us manage harmful outcomes and protect our well-being.