We recognize atmospheric pressure as a fundamental force driving storm development. Low-pressure systems draw in surrounding air, leading to cloud formation and precipitation, while high-pressure zones bring clear skies. Steep pressure gradients create strong winds, intensifying storms through mechanisms like cyclogenesis. Barometers measure these pressure changes, providing vital data for weather predictions. For instance, Hurricane Katrina's central pressure dropped to 902 mb, precipitating its destructive power. By tracking pressure patterns, we can predict storm intensity and path. Understanding these dynamics is essential, and further exploration will reveal even more intricate details of atmospheric interactions during storm events.
Key Points
- Atmospheric pressure variations influence the formation and intensity of storms.
- Low-pressure systems are associated with clouds, precipitation, and intense weather.
- Steep pressure gradients create stronger winds and more powerful storms.
- Accurate measurement of atmospheric pressure is crucial for storm prediction.
Basics of Atmospheric Pressure
Understanding atmospheric pressure is vital for comprehending how different weather patterns, including storms, develop. Atmospheric pressure, the force exerted by the weight of air above us, varies across different regions and altitudes. These pressure variations are pivotal in determining weather patterns.
When we examine atmospheric pressure, we notice that it's influenced by temperature, altitude, and the density of air molecules. In regions where the air is warm, molecules move more rapidly and spread apart, causing lower pressure. Conversely, cooler areas have denser air, leading to higher pressure. These differences create pressure gradients that drive air movement, shaping our weather.
High-pressure systems often bring clear skies and calm weather, while low-pressure systems are associated with clouds, precipitation, and storms. Meteorologists use barometers to measure atmospheric pressure and track these variations. By analyzing data over time, we can predict shifts in weather patterns. This understanding empowers us to anticipate and prepare for adverse weather conditions, ensuring our safety and freedom.
Pressure Gradients in Storms
High and low-pressure systems interact through pressure gradients, which are the driving forces behind storm formation and intensity. When we examine these gradients, we notice that the difference in atmospheric pressure between two regions dictates how wind patterns develop.
Air naturally moves from high-pressure areas to low-pressure areas, and this movement creates the winds that play an important role in storm dynamics.
As pressure gradients steepen, wind speeds increase, leading to more powerful and potentially destructive storms. For instance, a significant pressure drop in a localized area can result in rapid storm formation, as the accelerating winds carry moisture and heat, fueling the storm's energy.
Data collected from weather stations consistently show that regions with sharp pressure gradients often experience more intense weather events.
Moreover, the Coriolis effect, caused by Earth's rotation, influences these wind patterns, causing them to spiral. This spiraling motion is vital in the development of cyclones and hurricanes.
By understanding these pressure gradients, we can better predict storm paths and intensities, giving us the freedom to prepare and mitigate their impacts.
High Vs. Low Pressure Systems
Low-pressure systems, characterized by ascending air, typically bring precipitation and unsettled weather, whereas high-pressure systems, with descending air, generally lead to clear skies and stable conditions.
When we examine these pressure systems, we notice that low-pressure regions, or cyclones, encourage air to rise and cool, leading to cloud formation and precipitation. This dynamic is pivotal in understanding weather patterns associated with storms.
Conversely, high-pressure systems, or anticyclones, involve descending air that warms as it compresses, inhibiting cloud formation and promoting clear, calm conditions. It's fascinating how these opposing pressure systems interact to shape our weather.
For instance, the boundary between high and low-pressure systems, often referred to as a front, is a hotspot for dramatic weather changes.
Pressure and Storm Intensity
The intensity of storms is directly influenced by the pressure gradient between high and low-pressure systems, with steeper gradients often leading to more severe weather events. When we observe significant pressure changes, we can anticipate stronger winds and increased storm formation. This gradient creates a force that drives air from high-pressure areas to low-pressure zones, fueling the storm's intensity.
Pressure patterns play a vital role in storm tracking. By analyzing these patterns, we can predict the path and strength of storms with greater accuracy. The rapid drop in atmospheric pressure, known as cyclogenesis, often marks the birth of intense cyclonic systems. As we track these systems, understanding the pressure fluctuations helps us forecast their development and potential impact.
To better grasp the relationship between pressure and storm intensity, consider the following:
- Steep pressure gradients: Stronger winds and more powerful storms.
- Cyclogenesis: Rapid pressure drops leading to storm formation.
Measuring Atmospheric Pressure
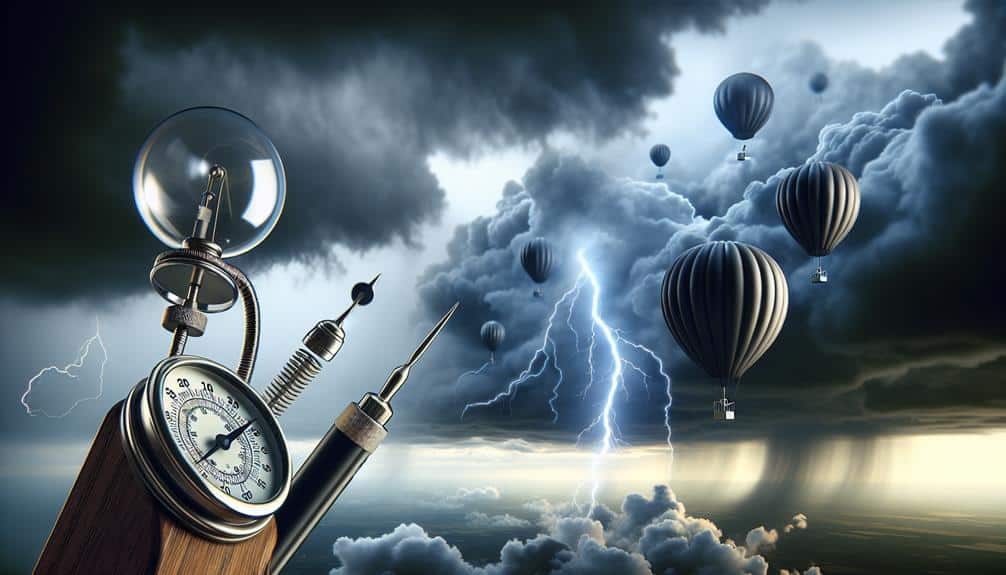
Let's examine how we measure atmospheric pressure using barometric instruments, various techniques, and ensuring calibration and accuracy.
Precise instruments like mercury and aneroid barometers are essential for accurate data collection.
Barometric Pressure Instruments
Accurate measurement of atmospheric pressure is achieved through instruments like barometers, which are essential for predicting weather and understanding storm formation. By monitoring barometric pressure, we can greatly enhance the accuracy of weather forecasting.
There are mainly three types of barometers: mercury, aneroid, and digital. Each type has its own advantages and unique calibration techniques, ensuring precise data collection.
Mercury Barometers: These use a column of mercury in a glass tube. Atmospheric pressure forces the mercury to rise or fall within the tube, providing a direct measurement. They require meticulous calibration but offer high precision.
Aneroid Barometers: These utilize a small, sealed metal box called an aneroid cell, which expands and contracts with pressure changes. They're portable and don't contain hazardous materials but need regular calibration to maintain accuracy.
Digital Barometers: These employ electronic sensors to measure pressure changes and often integrate seamlessly with modern weather forecasting systems. Their calibration is typically automated, promoting user-friendly operation.
Pressure Measurement Techniques
When measuring atmospheric pressure, we utilize precise calibration techniques and advanced technologies to guarantee the data's reliability and accuracy. We employ state-of-the-art pressure sensor technology, which includes electronic barometers, aneroid barometers, and piezoelectric sensors. These devices convert atmospheric pressure into an electrical signal, allowing us to capture real-time data with high precision.
We also leverage advanced data interpretation techniques to analyze the raw data collected from these sensors. This enables us to identify trends, anomalies, and patterns that are crucial in understanding atmospheric conditions. By using statistical models and computational algorithms, we can filter out noise and ensure the data is both accurate and actionable.
Our approach is rooted in the need for freedom in scientific exploration. We prioritize methods that minimize human error and maximize data integrity, ensuring our findings are reliable.
The integration of sophisticated pressure sensor technology with robust data interpretation techniques not only enhances our understanding of atmospheric pressure but also equips us with the tools to predict storm development more effectively. This thorough approach empowers us to make informed decisions, enhancing our ability to anticipate and respond to atmospheric events.
Calibration and Accuracy
How do we ensure the calibration and accuracy of our atmospheric pressure measurements?
Maintaining precise instrument calibration is vital for high data accuracy, which directly impacts our data interpretation and forecasting capabilities. We rely on several meticulous steps to uphold the integrity of our measurements.
– Regular Calibration:
We routinely calibrate our barometers using standardized reference instruments. This step is essential to correct any deviations and uphold data accuracy.
– Environmental Control:
We keep our instruments in stable environments. Factors like temperature and humidity can affect readings, so controlling these variables helps maintain consistency.
– Data Verification:
Cross-referencing data from multiple sources ensures our measurements are reliable. We validate readings with other stations and historical data to identify any anomalies.
Case Studies of Major Storms
In examining the role of atmospheric pressure in storm development, we'll analyze key data from major storms like Hurricane Katrina and Superstorm Sandy to understand their dynamics.
By scrutinizing the pressure patterns during these events, we can better comprehend how such storms evolve and intensify.
Hurricane Katrina, for instance, demonstrated rapid intensification due to a steep drop in central pressure, reaching 902 mb at its peak. This low pressure facilitated strong cyclonic activity and high wind speeds, driving catastrophic storm surges.
The storm's dynamics were markedly influenced by the warm Gulf waters and the pressure gradient between the hurricane's eye and the surrounding atmosphere.
Similarly, Superstorm Sandy showcased unique pressure patterns, merging with a mid-latitude trough that resulted in an expansive low-pressure system. The central pressure of Sandy dipped to 940 mb, amplifying wind fields and expanding the storm's reach.
This hybrid nature, combining tropical and extratropical characteristics, highlighted the complexity of storm dynamics influenced by varying pressure systems.
Frequently Asked Questions
How Are Atmospheric Pressure Changes Detected by Animals?
We've observed that animals exhibit specific behaviors in response to pressure changes. They possess barometric sensing capabilities, allowing them to detect shifts in atmospheric pressure, which triggers instinctive actions like seeking shelter or altering migration patterns.
What Role Does Temperature Play in Atmospheric Pressure Changes?
Temperature fluctuations directly affect pressure gradients. As temperature rises, air expands, decreasing pressure. Conversely, cooling air contracts, increasing pressure. These changes create pressure gradients, driving wind and influencing weather patterns, giving us a dynamic atmosphere.
Can Atmospheric Pressure Influence Human Health?
We've seen that barometric pressure changes can influence our health. Rapid drops in barometric pressure are linked to headaches and other weather-related health impacts. It's crucial to stay informed and understand these dynamics for our well-being.
How Does Atmospheric Pressure Affect Flight Operations?
How does atmospheric pressure affect flight operations? We prioritize flight safety, but low pressure can increase turbulence. Aircraft performance depends on pressure, affecting altitude restrictions. We adjust for these variables to guarantee smooth, safe flights for everyone.
What Technological Advancements Improve Atmospheric Pressure Measurement?
We've seen significant improvements in atmospheric pressure measurement with advancements in satellite technology and barometric sensors. These innovations provide us with precise, real-time data, enhancing our ability to predict weather patterns and ensuring our freedom to plan accordingly.