We explore supercell storm formation by analyzing wind shear's role in enhancing storm rotation, buoyancy-driven convection, and updraft strength in unstable atmospheres. Using Doppler radar, satellite imagery, and weather balloons, we quantify critical variables. Advanced computational models and real-time data from storm chasing missions provide valuable insights. Firsthand observations and in-situ measurements refine our predictive models and forecasting techniques. Additionally, mobile Doppler radars and high-resolution satellite imagery support real-time analysis. Future research leveraging machine learning and neural networks promises further advancements in predicting supercell behaviors influenced by climate change. For deeper insights, our thorough study offers detailed findings.
Key Points
- Doppler radar and satellite imagery provide critical data for identifying supercell formation and storm dynamics.
- Expert storm chasers offer invaluable first-hand observations and in situ measurements of supercell behavior.
- Computational models predict supercell dynamics using empirical data from weather balloons and ground sensors.
- Machine learning and neural networks enhance predictive models for supercell storm behavior.
Understanding Supercell Dynamics
To understand supercell dynamics, we must analyze the intricate interactions between wind shear, updraft strength, and atmospheric instability through empirical data and computational models. These elements are vital to comprehending how storm rotation develops and sustains itself.
Wind shear, defined as the change in wind speed and direction with height, provides the necessary environment for rotating updrafts. Strong wind shear allows for the horizontal vorticity to tilt and stretch, thereby enhancing storm rotation.
Atmospheric conditions, including temperature gradients and moisture levels, directly influence updraft strength. A potent updraft, which is essential for the formation of a supercell, arises from the buoyancy generated in an unstable atmosphere. When warm, moist air at the surface interacts with cooler, drier air aloft, it creates the instability needed for robust convection.
Empirical data from weather balloons, Doppler radar, and satellite imagery help us quantify these variables, while computational models simulate their interactions. By integrating real-world observations with advanced simulations, we can predict and analyze the behavior of supercells more accurately. This scientific approach empowers us to understand these powerful storms, enabling better forecasting and risk mitigation strategies.
Tools and Techniques
Leveraging advanced tools and techniques like Doppler radar, weather satellites, and computational fluid dynamics enables us to dissect the complex processes involved in supercell storm formation. By utilizing these methodologies, we can gain a more precise understanding of storm dynamics and behavior. Our primary focus remains on radar analysis and storm chasing, which provide invaluable real-time data.
- Doppler Radar: By measuring velocity patterns within the storm, Doppler radar aids in identifying rotational characteristics that signify the potential for supercell formation. It's essential for detecting mesocyclones, the hallmark of supercells.
- Weather Satellites: High-resolution satellite imagery allows us to monitor cloud structures and temperature profiles. This data is critical for observing the broader atmospheric conditions that contribute to storm genesis.
- Computational Fluid Dynamics (CFD): CFD simulations offer a detailed visualization of airflow patterns and pressure gradients within supercells. These models help us predict storm evolution and potential severity with greater accuracy.
- Storm Chasing: Direct on-ground observations provide empirical data that complements our radar and satellite analyses. It's a hands-on approach that enriches our understanding of supercell characteristics.
Expert Field Experiences
Drawing from years of on-the-ground research, we analyze how firsthand observations during storm chasing missions have enriched our understanding of supercell dynamics and behavior. By engaging directly with storm systems, we've gathered vital data that static instruments can't provide. Our meteorological observations during these missions have revealed intricate details about updraft rotation, mesocyclone formation, and tornado genesis.
As we navigate the turbulent conditions of a supercell, we utilize advanced radar and mobile weather stations to capture real-time data on storm structure and evolution. These in situ measurements allow us to track rapid changes in wind speed, humidity, and temperature gradients, which are essential in understanding the lifecycle of supercells.
The correlation between visual storm attributes and raw meteorological data has been pivotal in refining predictive models. Experiencing these phenomena firsthand not only enhances our theoretical knowledge but also sharpens our analytical skills. We've observed that supercells often exhibit unique behaviors not fully captured by remote sensing alone.
This direct engagement with the storms fosters a deeper comprehension of the variables at play, empowering us to develop more accurate forecasting methods and ultimately granting us the freedom to navigate and mitigate severe weather impacts effectively.
Data Collection Methods
We employ a combination of mobile Doppler radar, GPS-equipped weather balloons, and ground-based sensor arrays to systematically gather high-resolution data on supercell storm activities. This approach allows us to capture extensive datasets that are important for understanding the intricate dynamics of these powerful storms.
- Mobile Doppler Radar: We use mobile Doppler radar units to conduct real-time radar analysis. These units are mounted on vehicles, allowing us to move quickly and position ourselves strategically for data collection during storm chasing.
- GPS-Equipped Weather Balloons: Launching weather balloons equipped with GPS and various meteorological sensors helps us gather vertical profiles of the atmosphere. These profiles are essential for understanding temperature, humidity, and wind patterns at different altitudes.
- Ground-Based Sensor Arrays: We deploy an array of stationary sensors to monitor environmental conditions at the surface level. These sensors measure variables such as wind speed, barometric pressure, and rainfall intensity.
- High-Resolution Satellite Imagery: We utilize satellite imagery to complement ground-based observations. This provides a broader context for the storm's development and movement.
These methods enable us to conduct detailed radar analysis and improve our storm chasing strategies, ultimately advancing our understanding of supercell storm formation.
Future Research Directions
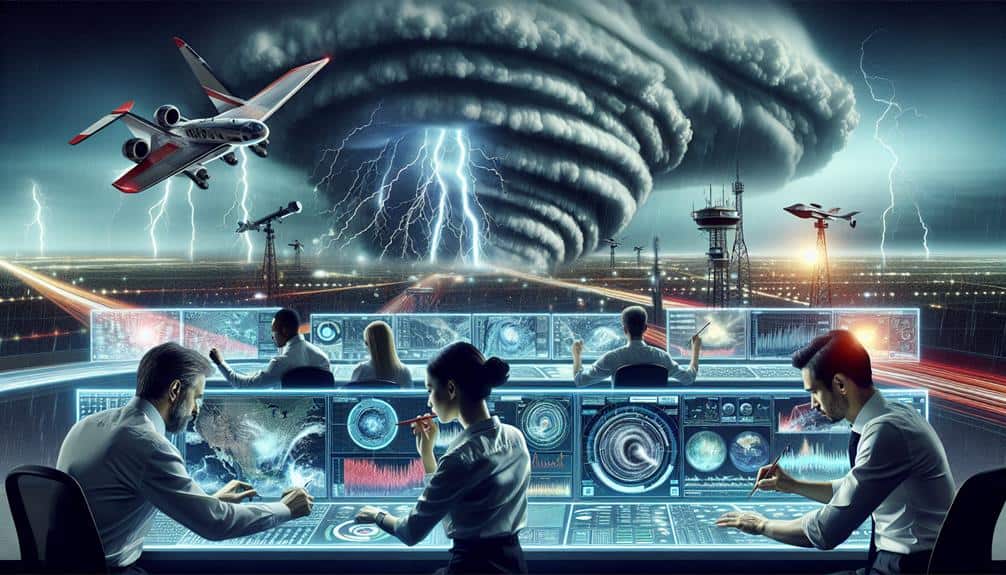
Building on our detailed data collection methods, our future research will focus on integrating machine learning algorithms to enhance predictive models for supercell storm behavior. By leveraging large datasets, we can train algorithms to identify patterns and anomalies in atmospheric conditions that precede supercell formation. This approach promises greater accuracy in storm prediction, enabling communities to better prepare and respond.
Our next steps include developing advanced neural networks that account for variables influenced by climate change. Rising global temperatures and shifting weather patterns complicate supercell dynamics, necessitating models that can adapt to evolving conditions. We'll use high-resolution climate simulations to feed our machine learning systems, ensuring that they remain robust under various future scenarios.
Moreover, we aim to collaborate with meteorological agencies to validate our models against real-world data. Implementing rigorous cross-validation techniques will refine our models' reliability and minimize false positives and negatives in storm prediction.
Frequently Asked Questions
What Are the Primary Safety Concerns When Researching Supercell Storms?
Dancing with nature's fury, we prioritize emergency preparedness and risk assessment. We rely on precise weather monitoring and meticulous data collection to guarantee our safety while capturing essential information, balancing our quest for knowledge with the freedom to explore.
How Do Supercell Storms Impact Local Wildlife?
Supercell storms drastically alter wildlife behavior and habitat impact, necessitating focused conservation efforts. Analyzing species resilience during these events reveals critical data for adaptive strategies, ensuring both ecosystems' health and our freedom to explore their complexities.
What Role Do Climate Change Trends Play in Supercell Storm Frequency?
Climate change implications, like a brewing storm, impact supercell formation. Our data shows rising temperatures and altered weather patterns increase frequency trends, intensifying storm activity. We must understand these shifts to mitigate future risks and preserve our freedom.
Can Supercell Storm Research Help Improve Early Warning Systems for Other Natural Disasters?
By improving early detection and disaster preparedness, supercell storm research enhances risk assessment and boosts community resilience. These advancements contribute to better early warning systems, ultimately empowering communities to maintain freedom and safety in the face of natural disasters.
How Are Findings From Supercell Storm Research Communicated to the General Public?
Think of our findings like a lighthouse guiding ships; we use data-driven communication strategies to enhance public awareness. We distill complex information into accessible formats, employ social media, and collaborate with media outlets for broader reach.